
Spacecrafts, particles and Lattice QCD
On March 2 1972, the Pioneer 10 space probe was launched from Cape Canaveral, Florida: direction Jupiter. Once completed its mission, this small spacecraft travelled towards the deep space, being the first artificial object to leave the solar system. Besides the 250 kilograms of scientific instruments, the probe contained also a peculiar piece of art: a message from humanity to alien civilisations, the so-called pioneer plaque. Being to possible to communicate with any language, this gold-anodized aluminium plaque, represented only pictures whose aim was to provide information about the origin of the spacecraft. There were representations of a male and a woman, of the probe itself, a map showing the position of the solar system and well as a picture of the planets composing it. Apart from it, in the top left corner the picture depicting the transition between two states of the hydrogen atom. Why? Because it is by far the most abundant element in the cosmos, the first to be studied by any civilisation. Richard Feynman , one of the most influent theoretical physicists of the 20th century, claimed
If, in some cataclysm, all of scientific knowledge were to be destroyed, and only one sentence passed on to the next generations of creatures, what statement would contain the most information in the fewest words? I believe it is the atomic hypothesis [...] that all things are made of atoms.
Richard P. Feynman, The Feynman Lectures on Physics
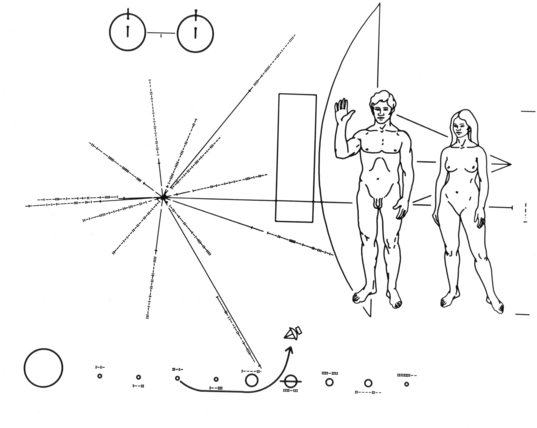
What is the atom made of? How many other particles are there?
All of you have heard about proton, neutron and electron at least once in your life. Indeed, these three particles constitute the atom as a whole: neutrons and protons bound together in a very small region of space (which is equivalent to one meter divided by 10 to the fourteenth power) and the electrons move around it in a region thousand times bigger than the nucleus itself.

These are probably the most widely known examples of particles, although there are many more and they differentiate essentially in two important categories: composite and elementary particles. While the former, if one looks close enough, contain other particles bounded together inside them, the latter do not hide a substructure. This means that they are not composed of smaller constituents.
There are seventeen elementary particles known up to now, and they have been artistically organised in the table below.
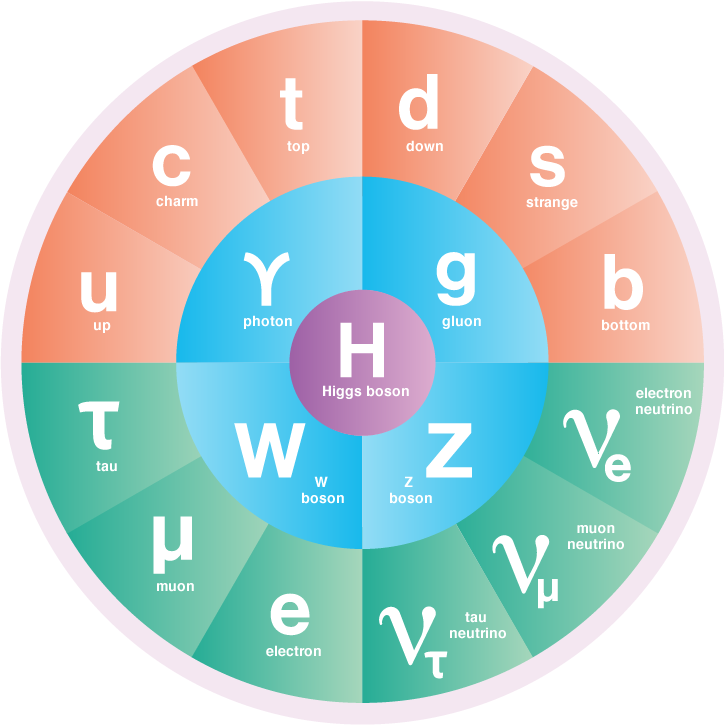
The picture was taken from https://www.symmetrymagazine.org/standard-model/
As you can see from the colours, they are divided into four main families:
Leptons (green): They are six and each of them possesses its own antiparticle. Leptons are subdivided into two subcategories, the first one is composed by electron, tauon and muon, while the second contains the neutrinos “counterparts” of these particles.
Quarks (red): As the leptons, they are six and each of them possesses its own antiparticle. There are two light quarks, up and down, that constitutes a huge percentage of the “ordinary matter”, i.e. they are contained in the atoms composing everything in the world; Then the fancy names come out: the remaining quarks are the strange, charm, top and bottom. The latter is much heavier than up and down, and they bound together giving heavier particles which usually are unstable and decay quickly.
Vector bosons (cyan): These particles are conceptually different from the latter since their role is to “mediate” interactions. What does it mean mediate? Well, let’s consider a simple example of two particles with opposite charges (positive and negative) put close to each other: our common experience says that they will attract each other. But how do they feel the presence of each other? By means of a vector boson, the photon! You can imagine this interaction as an exchange of photons between the two charges and in this sense, they “carry” the interaction.
There are mainly four vector bosons corresponding to three forces: apart for the photon, there are the W and Z bosons which carry the weak interaction and are responsible for radioactive decays, and the gluon which mediates the strong interaction acting on a subatomic scale binding the quarks inside the nuclei.
Last but not least, gravity: as far as we know, is a fundamental force but there not exist yet a theory explaining it from a microscopical point of view, in terms of vector bosons. This is probably one of the biggest open problems in physics.
Scalar bosons (purple): Last but not least, the famous Higgs boson. You have probably head about it, because of the fact that it has been theoretically predicted a long time ago and in 2012 experimental physicist s finally claimed they revealed the first signal supporting its existence. The Higgs boson interacts with many elementary particles, like for instance the electron, and through this interaction, it gives them a mass.
Starting from these elementary constituents, hundreds of different particles can be built.
At this stage you may think the physicist as someone collecting boring data about all these tiny particles in huge tables, to organise them like a stamp collector. Is this the real point of conducting research in particle physics?
The answer is at the same time simple and ambitious: finding a big scheme explaining everything, a definitive theory able to explain every phenomena in nature starting from the microscopical scale, predicting the way particles interacts among themselves, moving towards huge distances, explaining the structure of the Universe, how it evolved starting from the Big Bang and why it keeps expanding. In other words, being a physicist means to try to put together all these phenomena, explaining them according to a common set of rules, the physical laws. Saying it with Feynman words:
Curiosity demands that we ask questions, that we try to put things together and try to understand this multitude of aspects as perhaps resulting from the action of a relatively small number of elemental things and forces acting in an infinite variety of combinations.
Richard P. Feynman, The Feynman Lectures on Physics

The picture was taken from https://physicsforme.com
Standard Model of particle physics
The most promising theory, attempting to give an explanation to all these phenomena is called “Standard model”. It is not a definitive theory, in the sense that it cannot explain every physical phenomenon observed in nature. The Standard Model is composed of different sub-theories, each one of them explaining one fundamental force: the electroweak theory was originally composed of two distinct theories, explaining electromagnetism and weak interaction separately. However, later on, Weinberg, Salam and Glashow discovered that the two kinds of interaction are really interconnected and that they can be explained altogether with a unified theory. For this reason, in 1979 they were awarded the Nobel Prize in Physics. The second theory is Quantum Chromodynamics (QCD) and explains the strong interaction between subatomic particles, how quarks bind together giving the proton or neutron, as well as many other composite particles.
QCD, why so difficult?
Even though QCD, as we know it nowadays, has been formulated in the early 70s, it is still a hot topic in research and many of its properties have to be discovered yet. The reason for this resides in the so-called confinement phenomenon. In order to understand what this means let’s take a step back and consider the case of the better-known electromagnetic interaction.
Consider again the example of the two charges, with opposite signs, set apart in the space. Probably many of you have studied in school that the force between the charges is described by the Coulomb interaction,
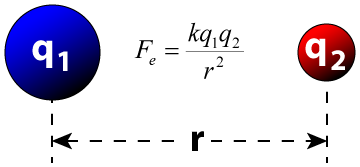
The picture was taken from https://www.toppr.com/
Which means that if we increase the distance between the bodies, the force decreases.
What if the force increases with the distance?
An example of such a scenario is familiar to all of us: if we put a rubber band around two fingers and try to set them apart, the bigger is the separation the stronger gets the force that obstacles our effort. Of course, the reality is much complicated, but this simple example helps to visualize how the force between quarks composing the proton acts. In particular if, for any reason, a quark and an antiquark are set apart the force that binds them increases with the distance separating the two particles. Moreover, like a rubber band that reached a threshold tension breaks, also the quark pairs may break giving rise to two quark pairs! This actually means that we can never observe a free quark, i.e. a not bounded with an antiquark.

The picture was taken from Wikipedia
The confinement phenomenon is a very fundamental physical property that affects how quarks bind together giving rise to composite particles, like the proton. It is quite remarkable the fact that, even though the confinement phenomenon is commonly recognized in the physical community, there is no mathematical proof of it yet! This means that, for instance, solving the equations of QCD for obtaining a description of the proton is not possible and other methods need to be employed in order to study these particles.
Beside some approximate theories that allow to approximately describe QCD in some particular situations, there was the need to change perspective in order to deeply understand the consequences of this fascinating theory. In 1974 Wilson proposed a new paradigm: instead of approaching QCD analytically one may try to simulate it. This idea, which was possible due to the increased computational power computers are capable of, was furtherly developed and refined during the years and allowed physicist to obtain high accurate physical predictions about QCD.
If you can't solve it...just simulate!
The basics idea behind these simulations is to rewrite the equations of Quantum Chromodynamics within a finite volume discretizing space and time. For this reason, this field of study is called Lattice QCD.
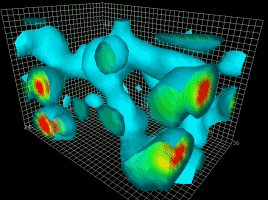
The picture was taken from http://www.physics.adelaide.edu.au
It is interesting to see how the volume that physicist has been able to simulate increased with the time, due to the speed-up and the improvement in memory of computational resources. In order to simulate QCD on large enough volumes, today physicist writes simulation software able to run on the most powerful supercomputers all over the world.

The picture was taken from https://www.olcf.ornl.gov/